By Mike Amaranthus and James M. Trappe
Abstract
Rapidly increasing CO2 levels in the atmosphere are resulting in abrupt changes in climate that threaten food production and ecological systems. Soils are crucial to managing climate change. They contain two to three times more carbon than the atmosphere. Arbuscular mycorrhizal fungi form a symbiotic relationship with the majority of our important crop species. How to capture dioxide from the atmosphere? A good opportunity is to “plant” carbon in soils and crops via arbuscular mycorrhizal fungi on millions of acres of farmland. Arbuscular mycorrhizal fungi and associated soil micro-organisms produce the sticky protein glomalin that results in vast CO2 sequestration and carbon storage in soil. Arbuscular mycorrhizal fungi also have the ability to directly absorb organic nutrients from soil and increase net primary productivity in stressful environments that significantly increases carbon accumulation during crop production. Managing soil to promote mycorrhizal fungi increases food production with less fossil fuel inputs than usual agricultural practice. Fostering arbuscular mycorrhizal activity and carbon deposition in soil are practical and economical now.
Introduction
Mushroom hunters are accustomed to keeping their heads down, looking for choice edible fungal prizes. Certainly, harvesting wild edible mushrooms can be a joyous and life-long endeavor. But sadly, only about 10% of earth’s several million fungal species produce mushrooms.
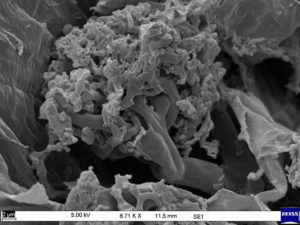
The majority not producing mushrooms, however, provide vital ecosystem functions. No fungal group is more important than the arbuscular mycorrhizal fungi. These tiny species are critical to life on earth. The term “arbuscular” is from Latin for “little bushes” referring to the microscopic fungal structures formed in host root cells in the shape of tiny bushes (Figure 1, left).
Arbuscular mycorrhizal fungi (formerly known as endomycorrhizal fungi) form a symbiotic relationship with most of our important crops as well as wild plants. Most grasses, wildflowers, agricultural plants, and woody plants form arbuscular mycorrhizae (Taber 1990, Trappe 1987, Wang and Qiu 2006). These host plants produce photosynthates to fuel the activities of arbuscular fungi colonizing their roots. The fungi, in return, pull nutrients and water from the soil for use by the host plants. Miles of arbuscular fungal filaments can be present in a tablespoon of healthy soil (Figure 2, below). Instead of producing specialized fruiting body structures such as mushrooms, truffles or puffballs with masses of spores, the arbuscular mycorrhizal fungi instead produce individual or clustered spores inside and outside the roots (Figure 3, below).
Figure 2: Arbuscular mycorrhizal fungi filaments
Figure 3: Arbuscular mycorrhizal spores
Arbuscular fungi dramatically increase the effective root systems of plants by producing a vast network of nutrient and water absorbing filaments. Farmers realize what goes on within the soil is as complex and important as that above the soil, although many may not be familiar with the important diversity of soil organisms. What we are beginning to appreciate is if managed correctly, these fungi can also sequester vast quantities of carbon from the atmosphere in an agricultural setting. The next big question in agriculture is really small: how can the many functions of arbuscular mycorrhizal fungi be applied to mitigate global problems?
The Dawn of Symbiosis
This salubrious phrase, coined by Bidartondo et al. (2011), refers to their insightful hypothesis on the early evolution of plant-fungus symbiosis. That term as translated from Greek literally means “living together” in English. Most, perhaps all, organisms rely on symbiosis with other organisms of one kind or another. If you are reading this, it is because of the symbiotic relationship between you and the bacteria in your gut! In his beautifully illustrated review of the literature on evolution of fungi and mycorrhizae, Halbwachs (2020) presents the evidence that spores of arbuscular mycorrhizal fungi (Glomeromycotina) and the earliest plant symbioses appear in the fossil record some 485 million years ago. The arbuscular mycorrhizal relationship turns out to be the most common form of root+fungus symbiosis and exemplifies an immensely successful product of evolution, best embodying Darwin’s phrase, “survival of the fittest.”
We Have a Problem
Agriculture spread dramatically some 12,000 years ago, and human-caused land clearings and crop tillage released substantial amounts of carbon dioxide into the atmosphere. Scientists using deep ice core analysis and techniques, detected early spikes in atmospheric carbon dioxide and methane that correspond to agricultural expansion thousands of years ago in Mesopotamia and China (Amundson et al. 2015).
More recently, since about 1750, with the rapid increase in the burning of fossil fuels and the 1900’s industrialization of agriculture, the scale and number of human-caused sources of carbon dioxide emissions have increased dramatically. With more carbon coming out of the ground now, and less returning to it, the level of carbon dioxide in the air is continuing to grow at a faster pace.
In the last 50 years atmospheric carbon dioxide levels have risen from 320 ppm to 415 ppm (Lindsey 2018). Usually, these kinds of changes in the atmosphere occur over geologic time scales. The effects on climate change are indisputable. Added CO2 has contributed to hotter and extreme changes in weather that are reducing crop production and making livestock more susceptible to disease. Acidification of the world’s oceans is threatening marine life. Climate change is threatening the health and safety of generations to come.
What If?
Carbon in the soil when released in the air combines with oxygen to become CO2, the greenhouse gas that contributes greatly to global warming. Common agricultural practices like plowing and tilling mix oxygen with soil carbon. Lately there has been tremendous attention examining reversing this process via carbon sequestration. The intent is to reduce CO2 levels in the atmosphere. Fifty years ago, few had heard of or cared about the concept. Carbon sequestration has suddenly become a hot topic.
Important questions are being addressed. How to best capture CO2 from the atmosphere? What organisms facilitate moving CO2 from the atmosphere to carbon in the soil, where it improves plant productivity? A good natural process is to enhance incorporating carbon into the soil via arbuscular mycorrhizal fungi on millions of acres of farmland (Figure 4, below). Research indicates that current agricultural practices release 25 to 35% of all carbon dioxide into the atmosphere, more than all types of transportation combined. Moreover, many farming practices such as intensive tillage and over-fertilization not only release carbon to the atmosphere but result in erosion and nutrient pollution of steams, lake, and oceans. But “what if” we could change farming from a destructive force to a regenerative force?
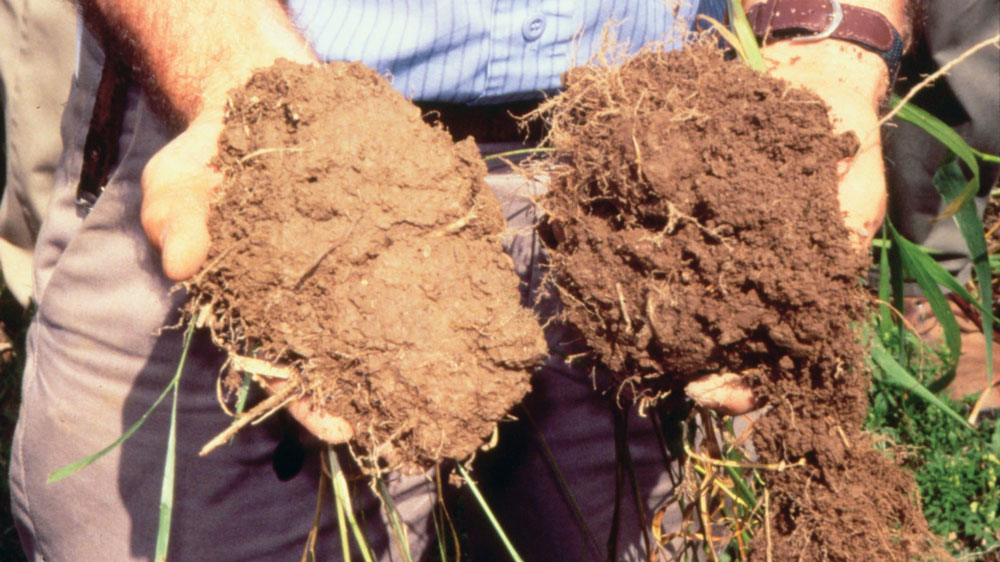
The knowledge and know-how for regenerative farming exist today. For example, we know that minimal or no tillage, fewer chemical fertilizers, and pesticides, cover cropping, and the addition of organic amendments are ways to increase soil carbon content, so less carbon dioxide is returned into the atmosphere and instead deposited in the soil (Pimental et al. 2005). Rates of carbon increase in the soil can exceed 1000 pounds per acre per year.
It’s not an expensive process to add carbon to soils. But it does take large numbers of people to participate. When managed appropriately on millions of acres of farmland, this approach can make a real difference in CO2 levels in the atmosphere. For example, increasing the carbon content of the world’s soils (outside of permafrost areas) by just a few parts per thousand (0.4%) each year would remove an amount of CO2 from the atmosphere equivalent to the fossil-fuel emissions of the European Union (around 3–4 gigatonnes (Gt) (Chabbi et al. 2017). It would also boost soil health: in studies across Africa, Asia and Latin America, increasing soil carbon by 0.4% each year enhanced crop yields by 1.3% (Soussana et al. 2019).
Governments are beginning to recognize the importance of adding carbon to soil. The Paris Agreement, also known as the Paris Climate Accord, is an agreement among the leaders of over 180 countries to reduce greenhouse gas emissions and limit the global temperature increase to below 2 degrees Celsius (3.6o F) above pre-industrial levels by the year 2100. The Paris Agreement includes the voluntary “4p1000 initiative”, which was launched under the framework of the Lima-Paris Action Plan in Paris on December 1, 2015. The name of the initiative reflects that a comparatively small proportional increase (0.4%) of the global soil organic carbon content in the top 0.3–0.4 m would store a significant amount of the global annual carbon emissions (Rumpel et al. 2018). The message is simple: increasing soil carbon can significantly mitigate carbon emissions and at the same time, adapt agricultural systems to climate change due to other numerous benefits. Soil carbon has positive effects on soil structure, water retention, and nutrient supply, and is crucial to sustain ecosystem services and agricultural productivity. What most people don’t realize is HOW this can happen? A key mechanism is through the keystone activities of arbuscular mycorrhizal fungi.
Key Players
Arbuscular mycorrhizae are the dominant mycorrhizal association on planet earth. They have been observed in the roots of more than 1,000 genera of plants representing some 200 families. It has been estimated that more than 80 to 90 percent of the estimated 400,000 plus species of vascular plants in the world form arbuscular mycorrhizae. These include most grains, vegetables, fruit and nut trees, vines and legumes.
With regard to stabilizing our increasingly unruly climate, mycorrhizal fungal biomass has been associated with the sticky carbon compound glomalin for hundreds of millions of years. Glomalin may be the most important soil component you have never heard of. Arbuscular mycorrhizal fungi play a key role in the carbon cycle by absorbing carbon that plant leaves fix in photosynthesis from the atmosphere. Carbon from the atmosphere is passed into the ground by the mycorrhizal fungi attached to the root system. These arbuscular mycorrhizal fungi are taxonomically included in the group Glomales, a major source of glomalin, and hence the name. Glomalin associated with endomycorrhizal fungal filaments is used to seal and gain enough rigidity to carry materials across the air spaces between soil particles. Bacteria associated with arbuscular mycorrhizal fungi are also a potential source of glomalin. Glomalin acts as a sticky organic glue that creates soil structure. Think of it as the material that holds the soil architecture together, allowing air, water, and roots to easily move through it. Without good soil structure, soils are vulnerable to soil erosion (Rillig et al. 2002). In addition, glomalin creates stable soil aggregates that protect soil carbon. Glued together by glomalin soil aggregates shelter organic matter rich in carbon and nutrients. The discovery is causing a reexamination of climate change modeling. Climate change models are rapidly incorporating new data on mycorrhizal fungi, glomalin and soil carbon storage into predictions of global warming rates. (Treseder et al. 2016).
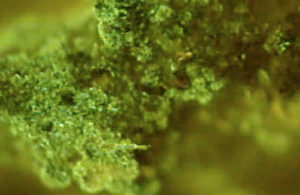
Mycorrhizal fungal activity has a key role influencing the huge pool of carbon in our soils. As much as 30 to 40 percent of the glomalin molecule is carbon. Glomalin may account for as much as one-third of the world’s soil carbon and the soil contains more carbon than all plants and the atmosphere combined! (Figure 5, left). While glomalin can last for decades and centuries in undisturbed soil, tillage and fallow can reduce it dramatically along with associated mycorrhizal fungi (Nichols and Millar 2013).
Other factors related to mycorrhizal fungi drive soil carbon accumulation and have the potential to mitigate climate change (Orwin et al. 2011). Organic nutrient uptake has been shown to significantly increase carbon accumulation in mycorrhizal fungal biomass, roots, and plants. Arbuscular mycorrhizal fungal filaments absorb organic nutrients in the form of amino acids (Whiteside et al. 2009); their study revealed that mycorrrhizal fungal filaments associated with Poa annua absorb carbon directly via the uptake of the amino acid glycine in the soil and was thereby transferred directly into plant leaves.
Mycorrhizal fungi also can increase carbon storage by improving the net primary productivity of cropping systems, especially systems limited by moisture or nutrients (Subramanian and Charest 1999). For example, mycorrhizal inoculation had a significant effect in improving water relations and in retaining more green leaf area, leaf water potential and stomatal resistance and transpiration rates in drought-stressed maize plants (Subramarian et al. 1995). Mycorrhizal fungi are key to crop growth and biomass production in stressful environments (Al-Karaki et al. 2004, Subramarian et al. 2006). (Figure 6, below). Mycorrhizal fungi might also be key to maintaining plant productivity and successful species migrations on rigorous sites during periods of climate change. (Perry and Amaranthus 1990).
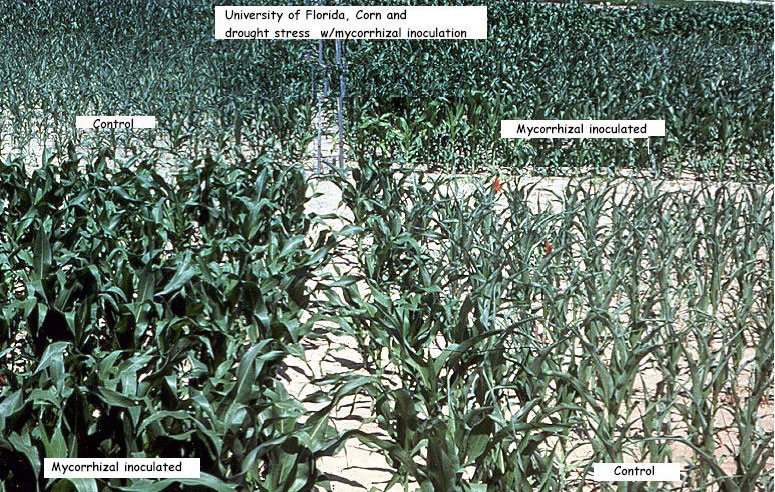
Squashing the Symbiosis
Unfortunately, many conventional agricultural practices reduce or eliminate mycorrhizal activity in the soil and release carbon dioxide into the atmosphere. Certain pesticides, chemical fertilizers, intensive cultivation, compaction, organic matter loss and erosion all adversely affect beneficial mycorrhizal fungi. An extensive body of laboratory and field-testing indicates that the majority of intensively managed agricultural lands lack adequate populations of mycorrhizal fungi (Douds et al. 1993).
Without the binding power of mycorrhizal filaments, soil structure deteriorates and a substantial amount of soil and carbon is eroded from surface soil (Amaranthus and Trappe 1993). Nutrients can be leached from the soil into waterways, where they can damage water quality and aquatic life. By destroying large segments of the soil food web, a large quantity of carbon and nutrient capital is lost, and the farmer is forced to add more fertilizer. Intensive cultivation and erosion damage the soil and mycorrhizal fungi, thereby releasing carbon to the atmosphere. Where the mycorrhizal association has been suppressed, mycorrhizal inoculation when planting crops with arbuscular mycorrhizal fungal inoculum is a cost-effective option for re-establishing the benefits of the symbiosis in the farmer’s field.
What We Know
The work of Rodale research collaborator, Dr. David Douds of the USDA Agricultural Research Service, suggests that healthy mycorrhizal fungi populations in organic systems increase crop productivity while sequestering carbon. Soils farmed with organic systems have greater populations of mycorrhizal fungi. Overwintering cover crops supply energy to fuel the activities of mycorrhizal fungi in the organic system, in contrast to the conventional systems, which generally have a significantly greater fallow period. Reduced chemical use in the organic system also provides an environment more favorable to the spread of the mycorrhizal fungi. Healthy populations of mycorrhizal fungi are associated with higher levels of glomalin production and increased carbon levels on farms. Yields can also increase. For example, in a 7-year study mycorrhizal fungi significantly increased vegetable production even in an existing high fertility environment (Douds et al 2016). Douds’ research suggests that a small amount of added mycorrhizal fungi can be substituted for a large amount of fertilizer in the growing of crops. The result: less CO2 emitted because of less fertilizer needs and less pollution of water resources because of fertilizer runoff.
Many of today’s farming practices can combine methods that increase carbon content in the soil. Instead of relying on synthetic pesticides and fertilizers, regenerative farming entails symbiotic and soil carbon-building practices such as growing legumes with associated nitrogen-fixing bacteria, cover crops, diversified crop rotations colonized with mycorrhizal fungi, and animal manures and compost (Pimental et al 2005). These are not pie-in-the-sky approaches but are proven ways to increase the carbon content of soil. What we need is more photosynthesis and practices that encourage life and carbon inputs in the soil. Healthy soils with vigorously growing plants are a carbon sponge.
Conclusions
For decades after World War II, massive inputs of fossil fuels, intensive tillage, chemical fertilizers and pesticides have helped increase crop productivity at the expense of topsoil erosion, organic matter loss, destruction of mycorrhizal fungi, and loss of precious soil carbon that turned to greenhouse gasses. These massive chemical inputs essentially covered up poor soil management practices. For three straight decades, carbon dioxide levels have skyrocketed, acres of productive farmland have declined, and farm input costs have increased. Where can we put carbon dangerously accumulating in the air? There is one practical and cost-effective approach — to put it back where it belongs, in the soil.
Much remains to be learned. What we don’t know about mycorrhizal fungi far exceeds what we do know. But one thing is becoming certain. Their effectiveness in sequestering carbon in the soil and reducing carbon emissions into the air cannot be overlooked. Considering mycorrhizal fungi also can significantly increase food production with less fossil fuel inputs makes their use a no-brainer Taking a fresh look at managing the carbon level in soils with mycorrhizal fungi has never made more sense.
Should we be trapped in a view of agriculture that is zero sum: agriculture wins and the environment loses? Agriculture loses and the environment wins? No, there is a better way. We need to manage our relationship with soil and agriculture so it can produce the food we need and at the same time increase soil fertility by imputing carbon into the ground. Can agriculture and mycorrhizal fungi mitigate climate change? The fungal game changer is hidden right beneath our feet.
Michael P. Amaranthus is a retired research soil scientist for the USDA and former associate adjunct professor at Oregon State University. He was the recipient of the USDA Highest Honors award for scientific achievement and has several mushrooms and truffles named in his honor. He is president of Myco Analytics LLC in Grants Pass, Oregon. drmikeamaranthus@gmail.com.
James M. Trappe is currently Professor, Forest Ecosystems and Botany-Plant Pathology at Oregon State University. With over 500 mycorrhizal scientific publications dating back since 1960 James continues his research activity today. Over the course of his career Jim has been author or joint author of 1 new order, 3 new families, 42 new genera/subgenera, 215 new species and 168 new species combinations. Jim has 18 fungi named in his honor.
Literature cited
Al-Karaki G., B. McMichael and J. Zak. 2004. Field response of wheat to arbuscular mycorrhizal fungi and drought stress. Mycorrhiza 14 (4): 263-269.
Amaranthus, M., and J. M. Trappe. 1993. Effects of disturbance on ecto-mycorrhizal and VA-mycorrhizal inoculum potential of soil in southwest Oregon. Plant and Soil 150(1): 41-49.
Amundson R., A. Berhe, J. Hopmans, C. Olson, A. Sztein, and D. Sparks. 2015. Soil and human security in the 21’st century. Science 348: 1261071.
Bidartondo M., D.J. Read, J.M. Trappe, V., Merckx, R. Ligrone, and J. G. Duckett. 2011. The dawn of symbiosis between plants and fungi. Biology Letters Aug 23; 7 (4): 574-577.
Comis D. 2002. Glomalin: Hiding Place for a Third of the World’s Stored Soil Carbon. Agricultural Research http://agresearchmag. ars.usda.gov/2002/sep/soil.
Douds D., R. Janke and S. Peters. 1993. VAM fungus spore populations and colonization of roots of maize and soybeans under conventional and low input sustainable agriculture. Ecosystems and Environment 43: 325-335.
Douds, D., J. Lindsay and C. Zieglar. 2016. Utilization of inoculum of AM fungi increases the yield of Solanum lycopersicum: A summary of 7 years of field trials on a conventional farm with high soil phosphorus. Scientia Horticulturae 207 (5): 89-96.
Halbwachs, H. 2020. Fungi in the rear mirror: a brief history of the fungi during the last two billion years. Fungi 13(2): 14-27.
Lal R. 2004. Soil carbon sequestration to mitigate climate change. Geoderma 123 (2): 1-22.
Lindsey, R. 2018. Climate change: atmospheric carbon dioxide. Climate.gov https://www.climate.gov/news-features/understanding-climate /climate-change-atmospheric-carbon-dioxide.
Nichols K., and J. Millar. 2013. Glomalin and soil aggregation under six management systems in the northern great plains, USA. Open Journal of Soil Science 3(8): 374-378.
Orwin, K., M. Kirschbaum, M. St John, and I. Dickie. 2011. Organic nutrient uptake by mycorrhizal fungi enhances ecosystem carbon storage. Ecology Letters 14(5): 493-502.
Perry D., and M. Amaranthus. 1990. Species migrations and ecosystem stability during climate change: the below-ground connection. Conservation Biology 4(30) 266-274.
Pimentel, D. P. Hepperly, J. Hanson, D. Douds and R. Seidel. 2005. Environmental, energetic, and economic comparisons of organic and conventional farming systems. Bioscience 55(7): 573-582.
Rillig, M., S. Wright, and V. Eviner. 2002. The role of arbuscular mycorrhizal fungi and glomalin in soil aggregation: comparing effects of five plant species. Plant and Soil 238: 325–333.
Rumpel C, F. Amiraslani, L-S Koutika, P. Smith, D. Whitehead and E. Wollenberg. 2018. Put more carbon in soils to meet Paris climate pledges. Nature 564:32-34.
Taber, R. 1990. Mycorrhizal fungi in wildflowers and weeds. Wildflower 4(2): 202-224.
Trappe J. M. 1987. Phylogenetic and ecologic aspects of mycotrophy in the angiosperms from an evolutionary standpoint. In: Ecophysiology of VA Mycorrhizal Plants, edited by Safir G. I. CRC Press, Boca Raton, USA: 525.
Treseder, K. 2016. Model behavior of arbuscular mycorrhizal fungi: predicting soil carbon dynamics under climate change. Botany 94(6): 417-423.
Treseder, K., and K. Turner. 2007. Glomalin in Ecosystems. Soil Science Society of America 71(4): 347-355.
Soussana, J., F. Lutfalla, F. Ehrhardt, T. Rosenstock, C. Lamanna, P. Havlík, M. Richards, E. Wollenberg, J. Chotte, E. Torquebiau, P. Ciais, P. Smith and R. Lal. 2019. Matching policy and science: Rationale for the ‘4 per 1000’ – soils for food security and climate’ initiative. Soil and Tillage Research 188. 3-15.
Subramanian K. and C. Charest. 1999. Acquisition of N by external hyphae of an arbuscular mycorrhizal fungus and its impact on physiological responses in maize under drought-stressed and well-watered conditions. Mycorrhiza 9(2): 69-75.
Subramanian, K.S., C. Charest, L. M. Dwyer and R.I. Hamilton. 1995. Arbuscular mycorrhizas and water relations in maize under drought stress at tasselling. New Phytologist 129:643 650.
Subramanian K., P. Santhanakrishnan and P. Balasubramanian. 2006. Responses of field grown tomato plants to arbuscular mycorrhizal fungal colonization under varying intensities of drought stress. Scientia Horticulturae 107: 245-253.
Wang, B., Y.L. Qiu. 2006. Phylogenetic distribution and evolution of mycorrhizas in land plants. Mycorrhiza 16: 299–363
Whiteside, M., K. Treseder and P. Attsatt. 2009. The brighter side of soils: Quantum dots track organic nitrogen through fungi and plants. Ecology 9(1): 100-108.